Perennial grain cropping systems provide many beneficial attributes for the environment as compared to their annual analogues . Perennial crops are more resource-use efficiency and contribute less to soil erosion. The domestication of the perennial Intermediate Wheatgrass (IWG) for grain and forage production is being developed and a semi-commercial variety is currently used (i.e. Kernza®) in USA and Canada. However, the effect(s) of domestication on the
Perennial grain cropping systems provide many beneficial attributes for the environment as compared to their annual analogues . Perennial crops are more resource-use efficiency and contribute less to soil erosion. The domestication of the perennial Intermediate Wheatgrass (IWG) for grain and forage production is being developed and a semi-commercial variety is currently used (i.e. Kernza®) in USA and Canada. However, the effect(s) of domestication on the


The monocot grass, Setaria viridis, is an emerging C4 model specie in the field of plant biology and biotechnology. Setaria viridis, is a diploid plant utilizing C4 photosynthesis. It belongs to the Poaceae family, subfamily Panicoideae, which comprises the most agronomical important grass crops such as the cereals maize, sorghum and millet. Members of the Panicoideae subfamily of grasses grow crown roots from their shoots a characteristic that is not observed in many well studied eudicots such as Arabidopsis and Tomato. While the crown roots sustained growth and branching allow optimal soil exploration, the hydraulic conductivity of those roots favors the radial transfer of water from the soil for subsequent transport to the shoot. However, the molecular and physiological mechanisms that regulate crown-root hydraulics are poorly understood. Aquaporins, which are water channel proteins that facilitate water transport across root cell membranes, could be important components of radial crown-root water transport. Although they represent a key point for the adaptation of wild plant species to diverse natural habitats and are a major target for crop improvement under climate change scenarios, little is known about the signaling mechanisms that link soil properties to crown-root hydraulics and aquaporin functions, particularly in grasses. Therefore, we will use two Panicoideae model plants, Setaria viridis accessions that differ in their crown-root traits and stress responses (Saha, Sade et al., 2016) to study the genetic and molecular mechanisms that control crown-root hydraulic conductivity and root-to-shoot gas-exchange signaling under variable environmental conditions. Our proposed study will aim to answer the following questions: What is the contribution of AQP to crown-root hydraulics and how does that contribution compare to the contribution of root morphology (i.e., xylem structure and development). What are the most prominent crown root-specific AQPs? What role (s) do these individual AQPs play in determining radial root hydraulics and the whole-plant gas-exchange rate? To what extent are crown-root AQPs involved in mediating the whole-plant response to drought? What are the genetic components that regulate crown-root hydraulics in grass plants under changing environmental conditions? To address these questions, we will employ the following approaches in crown-root tissue from the two S. viridis accessions: (i) transcriptome analysis to determine the prominent crown-root AQP, (ii) analysis of crown-root cell water permeability (using protoplasts) and AQP activity (using AQP blockers), (iii) anatomical (cross-sectional) and developmental (in situ root imaging) analysis of crown roots, (iv) analysis of crown root to shoot gas-exchange signaling (LI-COR and PlantDitech), (v) crown root-specific overexpression and knockout of an AQP, (vi) genomic and transcriptomic comparison of the two S. viridis accessions and (vii) generation of recombinant inbred lines (RILs) and a genetic map for the identification of SNPs and genes associated with crown-root traits. This project will yield unique information on molecular and genomic changes related to root and whole-plant water use and stress tolerance, and the research findings will be a valuable resource for grass research and improvement of grass crops. This work will highlight the importance of water conductivity in different types of roots and will expand our knowledge of the molecular basis of aquaporin-mediated stress signals, root hydraulics and whole-plant transpiration in grasses. Furthermore, this study will also yield a wealth of new, high-quality data regarding root tissue-specific optimal and stress-related gene-expression profiles for the model plant Setaria viridis.


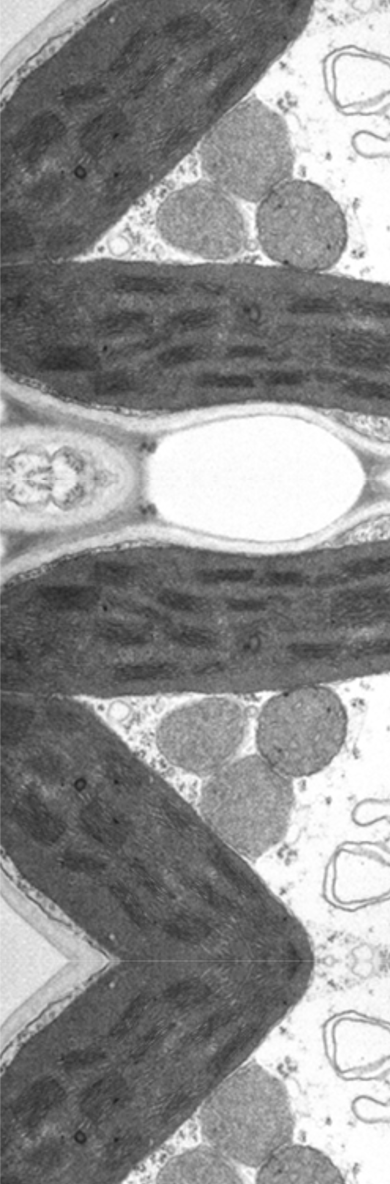


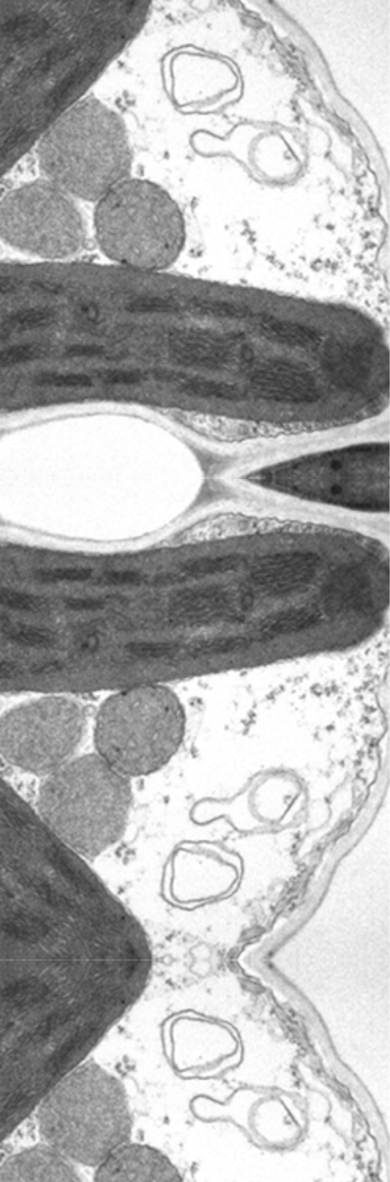
Developing perennials grains for a sustainable agriculture in a changing climate
​
Perennial grain cropping systems provide many beneficial attributes for the environment as compared to their annual analogues . Perennial crops are more resource-use efficiency and contribute less to soil erosion. The domestication of the perennial Intermediate Wheatgrass (IWG) for grain and forage production is being developed and a semi-commercial variety is currently used (i.e. Kernza®) in USA and Canada. However, the effect(s) of domestication on the response(s) to abiotic stress of Kernza is not understood and the feasibility of expanding the growth of IWG to Mediterranean conditions (i.e. hot summers) has not been tested. The overall objective of this research is to identify physiological and biochemical traits for breeding perennial grain crops able to growth under Mediterranean-like stress conditions. We will compare the responses of domesticated and non-domesticated perennial IWG varieties grown under optimal and abiotic stress conditions to identify traits associated with the stress response and traits that are associated with crop domestication. We will apply a Systems Biology approach, combining physiology, biochemistry, genomics, metabolomics and bioinformatics to identify genes and pathways that will define useful traits to crop breeders. The development of stress-tolerant perennial grain varieties is an important priority in the present scenario of climate change, soil erosion and diminishing food security. The outcome of the research will greatly contribute to the identification of key traits associated with sustainable growth and enhanced yield of perennial grains, growing under stress conditions that are typical to Mediterranean climates. In addition, key traits identified in the work done on the commercial KERNZA (e.g., N/C homeostasis, stress induced chloroplast degradation and water relations) will be functionally characterized using using gain-of-function (gene expression under the control of stress-induced promoters) and loss-of-function (gene silencing and gene editing – CRISPR/Cas 9) approaches in a perennial grass model plant Brachypodium Sylvaticum. Brachypudium sylvaticum is a perennial grass that is a suitable model for the study of perennial grass. We have studied the abiotic stress tolerance of WT accessions using physiology (whole plant measurements) cell biology (e.g cell and tissue sodium transport), genomics (RNAseq) and biochemistry (metabolomics and lipidomics) and identify suitable genetic material for the study of perennial crops response to climate change.
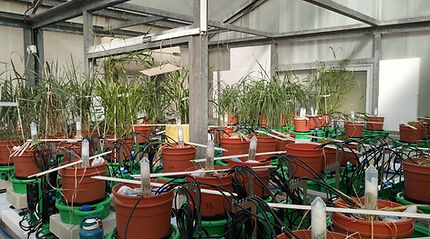

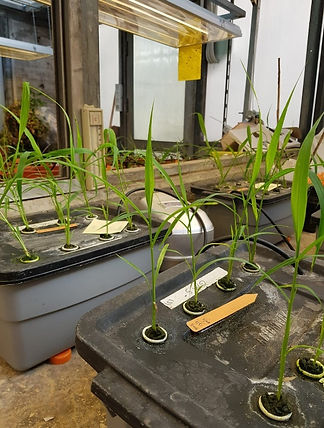


Who We Are
​
A systems-biology approach to elucidating the metabolic pathways for the abiotic-stress tolerance of wild emmer wheat
​
One major focus in agriculture today is enhancing crop productivity under stress conditions (e.g., drought, heat and salinity). This is a major concern due to many factors, including intensifying climate change (e.g., changing rain and evaporation patterns), anthropogenic desertification (due to grazing and the overuse of existing agricultural habitats) and the increasing human population with a rising standard of living. One way towards solve this snowballing problem is to discover genes and pathways associated with abiotic-stress tolerance. Wild relatives of crop plants are a rich source for novel stress-resistance genes. Since wild plants have been dealing evolutionarily with biotic and abiotic stresses for millennia, their genomes contain many genes related to resistance, which can be introduced into domesticated crop species. Traditionally, this has been done through wide crossing andvigorous and continuous breeding schemes. In the past few decades, plant transgenesis has facilitated this process tremendously, saving time, money and resources. In the past 20 years, the Omics age has emerged, allowing the relatively cheap generation of high-throughput data in genomics and metabolomics. One major setback to this revolution is the human inability to manually process this much data. Therefore, many are turning to statistics and computational biology to bridge this data-analysis gap. In this project, we are utilizing the unique genetic materials of the Institute for Cereal Crop Improvement (ICCI) and a Systems Biology approach that brings together traditional physiological experiments with Omics (i.e., genomics, metabolomics and microbiomics) involving computational and statistical methods (e.g., machine learning and correlation network analysis) to uncover previously unknown genes and pathways associated with resistance to drought and salinity stress in wild and domesticated wheat.
​
​
Improving tomato yield and fruit quality by enhancing source/sink relationships through the regulation of chloroplast stability
Physiological and molecular mechanisms controlling root hydraulic conductivity and root-to-shoot gas-exchange signaling in Setaria viridis under variable environmental conditions
​
​
Chloroplast are the main source for production of both carbon (C) and nitrogen (N) in plants. Several pathways are involved in the regulation of source/sink capacity and C/N assimilation both under well water and stress conditions: (I) CELL WALL INVERTASE (CWINV) will enhance sink capacity and carbon fluxes from leaves to fruits; (II) CHLOROPLAST VESICULATION (CV) can degrade chloroplasts, accelerating C- and N-allocations to the sinks (Sade et al., 2018b) and (III) Inhibition of CV under stress will delay stress induced chloroplast degradation and enhance C/N assimilation and source capacity. Increasing the C and N assimilation during vegetative growth and stress episode(s) and increasing the C/N allocation to the fruit during development and plant senescence are both beneficial traits for fruit quality. Although both traits can potentially increase fruit production and quality, they are potentially antagonic unless they are timed correctly and research aimed at their expression, but avoiding deleterious effects, is required. Moreover, a better understanding of the genetic components that control C/N allocation especially under stress is needed. Our hypothesis is that the combination of (i) enhancing the source C/N capacity throughout the growth season and stress episode and (ii) maximizing the C/N source to sink allocation at fruit development will result in higher fruit productivity and better fruit quality. Thus, while enhancing source C/N capacity and source to sink allocations will enhance plant growth and development, the induction of CV in the source leaves and CWINV in fruits - during fruit maturation – will increase sugar loading in the fruit (and increase the fruit %Brix). We will therefore design and transform tomato plants with multiple genes constructs comprising two different genes cassette: 1) CRISPR/Cas 9 system targeting SlCV for the generation of knockout; this will enhance the source capacity during stress and 2) DEX::CV (Chemically induced expression of CV; that is not targeted by CRISPR/Cas 9) for synchronized activation of CV during fruit maturation and increased C/N allocation. Moreover, those transgenic plants will be generated on the background of a near isogenic line IL9-2-5 which have a strong CWINV fruit activity and presented enhance sink capacity. In addition to the detailed characterization of the transgenic plants source/sink relationship and yield component, those plants will be further studied using system biology approach to better understand the molecular and genetic components that regulate the allocation of C/N from source to sink (e.g., sugar and amino acid transporters)
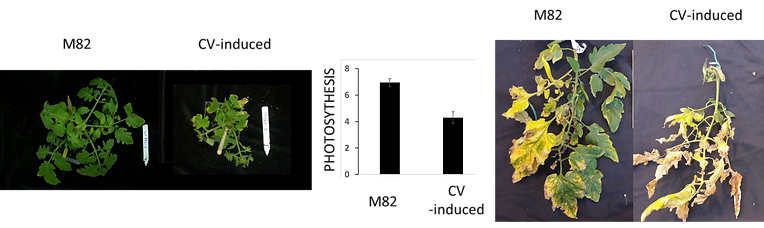
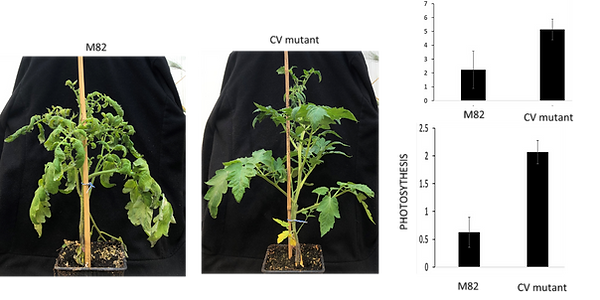
Grasses (e.g., the Panicoideae subfamily, which is particularly important for agriculture) sprout crown roots from their shoots. While the sustained growth and branching of those roots allows for optimal soil exploration, the water permeability of those roots (in other words, root hydraulic conductivity) favors the radial transfer of water from the soil for subsequent transport to the shoot. Nevertheless, the molecular and physiological mechanisms that regulate root hydraulics are poorly understood. Aquaporins (AQPs; water-channel proteins) facilitate the transport of water across root cell membranes. AQPs could be main effectors of radial crown-root water transport (alongside other as yet unknown regulators). Although they represent a key point for the adaptation of wild plant species to diverse natural habitats and are a major target for crop improvement, the signaling mechanisms that link soil properties with root hydraulics and AQP functions remain largely unknown, especially in grasses. The main goal of this project is to gain better understanding of the physiological and molecular mechanisms controlling root hydraulic conductivity and root-to-shoot gas-exchange signaling (e.g., water use efficiency). The work will be conducted in the model cereal plant Setaria viridis under variable environmental conditions. Using physiological (i.e., gas exchange and root hydraulics), molecular (i.e., gene editing, expression analysis), biochemical (i.e., AQP inhibitors, protein‒protein interaction) and cellular (i.e., cell water permeability and cell localization) tools, we have identified a unique AQP (designated PIP1;6) which is a candidate for the regulation of root hydraulics and shoot gas exchange

Physiological and biochemical traits associated with domestication and the effects of climate change on a perennial cereal grain
complicated genome, phenotyping is still very helpful in revealing those plant traits. High-throughput phenotyping (HTP) techniques are advanced and novel tools for evaluating the physiological traits of complex plants. We have set up cutting-edge HTP physiological systems for theaccurate and precise characterization of water-use efficiency. Those systems include a gravimetric-based system (known as Plant-Ditech) and a gas-exchange system (known as LICOR6800). We have integrated the experimental setup and data analysis for those systems, to be used in our own research as well as by other
the domestication of IWG has accelerated, mainly toward high yield and other important agronomic traits. Nevertheless, studies in other cereals have shown that domestication has a negative impact on plant resilience to abiotic stress, especially on water-use efficiency. Due to increasing extreme weather events that are exacerbating world food insecurity, it is crucial to understand and enhance the abiotic-stress tolerance of IWG. Due to IWG’s
members of the department (see intra-departmental collaboration). In this study, we analyzed how the domestication of IWG has influenced the plant’s physiological behavior in response to Mediterranean abiotic stress, specifically drought stress. Using continuous data from the HTP regarding the plant’s water management and growth in a semi-controlled environment together with other physiological data, we identified different trends between plants’ breeding cycles. It seems that the last cycle of domestication reduced its shoot/root ratio in reaction to drought. In contrast, during earlier cycles, a high shoot/root ratio was maintained.
Intermediate wheatgrass (Thinopyrum intermedium; IWG) is one of the promising perennial grains for sustainable agriculture. The cultivation of perennial grains has been suggested as a complementary strategy to the cultivation of traditional annual grains. Reduced soil erosion, nitrogen leakage and carbon dioxide emissions are a few of the environmental advantages of perennial grains over annual grains. In the last decade,


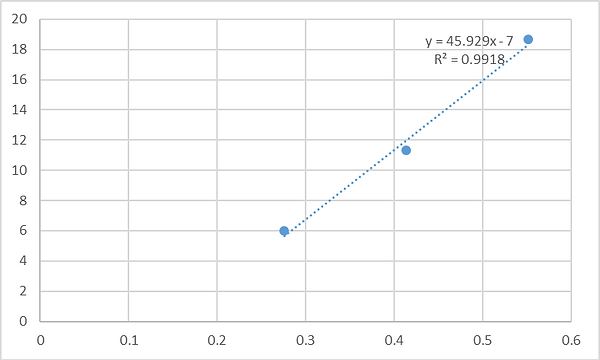